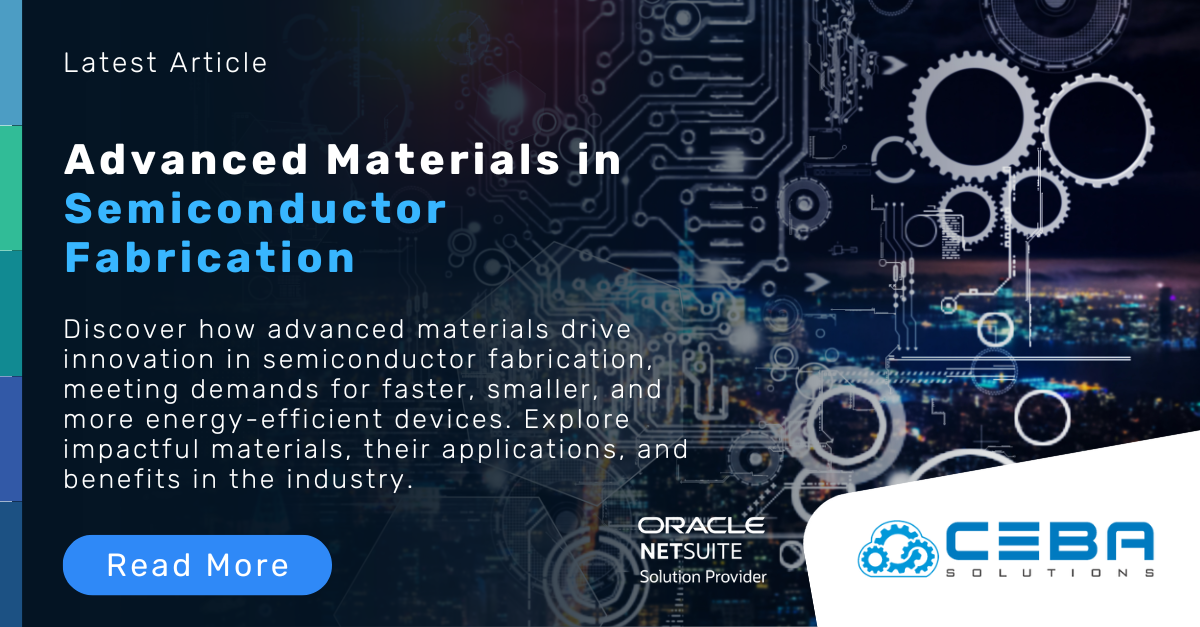
Advanced Materials in Semiconductor Fabrication
Advanced Materials in Semiconductor Fabrication
The semiconductor industry is the backbone of modern technology, enabling the development of everything from smartphones and computers to advanced medical devices and autonomous vehicles. As the demand for faster, smaller, and more efficient electronic devices grows, the semiconductor industry is continually evolving to meet these challenges. One of the key drivers of this evolution is the development and integration of advanced materials in semiconductor fabrication. These materials are crucial for enhancing performance, reducing power consumption, and enabling new functionalities. This article explores the role of advanced materials in semiconductor fabrication, highlighting their benefits, applications, and future trends.
The Role of Advanced Materials in Semiconductor Fabrication
Semiconductor fabrication involves the creation of integrated circuits (ICs) on semiconductor wafers. This process requires materials with specific electrical, thermal, and mechanical properties. Advanced materials play a critical role in overcoming the limitations of traditional silicon-based technologies, enabling the production of more powerful and efficient devices. Here are some key areas where advanced materials are making a significant impact:
High-K Dielectrics
High-K dielectrics, such as hafnium oxide (HfO2), are used as gate insulators in transistors. These materials have a higher dielectric constant than silicon dioxide (SiO2), allowing for improved capacitance and better control of the transistor channel. This improvement in capacitance is essential for scaling down transistor sizes while maintaining performance. Additionally, high-K dielectrics help reduce gate leakage currents, which is crucial for enhancing the performance and power efficiency of modern transistors. By mitigating leakage, these materials contribute to lower power consumption and extended battery life in portable devices.
Metal Gate Electrodes
The transition from traditional polysilicon gates to metal gate electrodes, such as titanium nitride (TiN) or tantalum nitride (TaN), represents a significant advancement in semiconductor technology. Metal gates offer superior conductivity and performance, essential for high-speed and low-power applications. Moreover, metal gate electrodes are critical for scaling down transistor dimensions, supporting the continued miniaturization of integrated circuits. This miniaturization is pivotal for increasing transistor density on a chip, thereby enhancing the computational power and efficiency of electronic devices.
Strained Silicon
Strained silicon involves stretching the silicon lattice to improve electron and hole mobility. This technique enhances the performance of transistors by increasing their switching speed and reducing power consumption. The increased mobility in strained silicon transistors results in higher drive currents, making them ideal for high-speed and high-performance applications. As a result, strained silicon is a key enabler for the development of faster processors and more efficient power management systems in various electronic devices.
III-V Semiconductors
III-V semiconductors, such as gallium arsenide (GaAs) and indium phosphide (InP), offer superior electron mobility and direct bandgap properties compared to silicon. These materials are used in high-frequency and optoelectronic applications, enabling the fabrication of advanced devices such as high-electron-mobility transistors (HEMTs) and laser diodes. These devices are essential for telecommunications and photonics, providing the high-speed and efficient operation required for modern communication systems and optical technologies.
Graphene and 2D Materials
Graphene, a single layer of carbon atoms arranged in a hexagonal lattice, exhibits exceptional electrical conductivity, mechanical strength, and thermal properties. It is being explored for use in transistors, sensors, and interconnects, potentially revolutionizing various aspects of semiconductor technology. Beyond graphene, other 2D materials, such as molybdenum disulfide (MoS2) and hexagonal boron nitride (h-BN), offer unique properties that could complement or even surpass silicon in certain applications. These materials are paving the way for the development of ultra-thin, flexible, and high-performance electronic devices.
Phase-Change Materials
Phase-change materials, such as germanium-antimony-tellurium (GeSbTe), are used in non-volatile memory technologies like phase-change memory (PCM). These materials can switch between amorphous and crystalline states, enabling data storage with high endurance and fast read/write speeds. PCM technology offers advantages in terms of speed and durability compared to traditional memory technologies, making it a promising candidate for future high-performance and high-capacity memory solutions.
Applications of Advanced Materials in Semiconductor Fabrication
Transistors and Logic Circuits
Advanced materials are crucial for the continued scaling of complementary metal-oxide-semiconductor (CMOS) technology, which is the foundation of most digital ICs. Materials like high-K dielectrics and metal gates are essential for fabricating advanced transistor architectures, such as Fin Field-Effect Transistors (FinFETs) and Gate-All-Around FETs (GAAFETs), which offer improved performance and scalability. These innovations enable the production of faster and more energy-efficient processors, driving the advancement of computing technologies.
Memory Devices
High-K materials and metal gates improve the performance and reliability of dynamic random-access memory (DRAM) and flash memory devices. Emerging memory technologies, such as PCM, resistive RAM (ReRAM), and magnetoresistive RAM (MRAM), are also benefiting from advanced materials. These new memory technologies offer better performance, energy efficiency, and endurance, addressing the growing demand for high-capacity and high-speed memory solutions in data-intensive applications.
Optoelectronics and Photonics
III-V semiconductors are used to fabricate light-emitting diodes (LEDs) and laser diodes for applications in lighting, displays, and optical communication. Advanced materials like graphene and III-V compounds are used in high-performance photodetectors for imaging and sensing applications. These materials enable the development of more efficient and high-resolution optical devices, contributing to advancements in fields such as telecommunications, medical imaging, and environmental monitoring.
Power Electronics
Wide bandgap semiconductors, such as silicon carbide (SiC) and gallium nitride (GaN), are used in power electronics for their high breakdown voltage, thermal conductivity, and efficiency. These materials are essential for applications in electric vehicles, renewable energy, and power management. By enabling more efficient power conversion and management, wide bandgap semiconductors contribute to the development of greener and more sustainable technologies.
Flexible and Wearable Electronics
Organic semiconductors and 2D materials like graphene are used in flexible and wearable electronics, enabling the development of lightweight, bendable, and stretchable devices. These materials support the creation of innovative electronic products, such as flexible displays, wearable sensors, and electronic textiles, opening new possibilities for consumer electronics and healthcare applications.
Future Trends and Challenges
Materials Innovation
Ongoing research is focused on discovering and synthesizing new materials with superior properties to push the boundaries of semiconductor performance. Combinatorial approaches, such as heterostructures and superlattices, can create devices with unique functionalities. These innovative materials and structures are expected to drive the next wave of advancements in semiconductor technology, enabling more powerful, efficient, and versatile electronic devices.
Integration and Compatibility
Integrating advanced materials into existing semiconductor fabrication processes without compromising yield and reliability is a significant challenge. Ensuring compatibility between different materials and minimizing defects at interfaces is crucial for device performance. Overcoming these challenges requires continuous innovation in fabrication techniques and process engineering to fully realize the potential of advanced materials.
Environmental and Economic Considerations
Developing environmentally friendly and sustainable manufacturing processes for advanced materials is essential for reducing the ecological impact of semiconductor fabrication. Balancing the cost of advanced materials with their performance benefits is critical for their widespread adoption in the semiconductor industry. As the demand for sustainable and cost-effective solutions grows, the industry must prioritize the development of greener manufacturing practices and materials.
Conclusion
Advanced materials are at the forefront of the semiconductor industry's efforts to meet the demands of modern technology. By enhancing performance, enabling new functionalities, and supporting the continued miniaturization of electronic devices, these materials are driving innovation and shaping the future of semiconductor fabrication. As research and development continue to push the boundaries of material science, the integration of advanced materials will play an increasingly vital role in the evolution of the semiconductor industry. Overcoming challenges related to integration, compatibility, and sustainability will be key to unlocking the full potential of these materials and ensuring the continued progress of high-tech electronics.